This article will explore the potential of Small Nuclear Reactors (SMRs) in mitigating climate change if they can save us from global warming.
Table Of Content
Nuclear power may seem like a last resort in a world where climate change poses an existential danger, but it’s really our best hope. Proponents of nuclear power point to the technology’s ability to replace fossil fuels and reduce carbon emissions all in one tidy package. However, the nuclear business is on the decline around the globe. Public mistrust persists, particularly in the wake of the 2011 catastrophe at Japan’s Fukushima Daiichi Nuclear Power Plant, in which three reactors burned down. In addition, countries remain undecided over how to deal with waste from nuclear reactors. Most importantly, the high price of new reactors ($7 billion or more) makes the nuclear business less competitive than its counterparts in the fossil fuel and natural gas industries. Only one nuclear reactor has been activated in the United States in the last 20 years, despite the world continuing to set temperature records. Today, nuclear power generates just 11% of the world’s electricity, down from 17% in 1996.
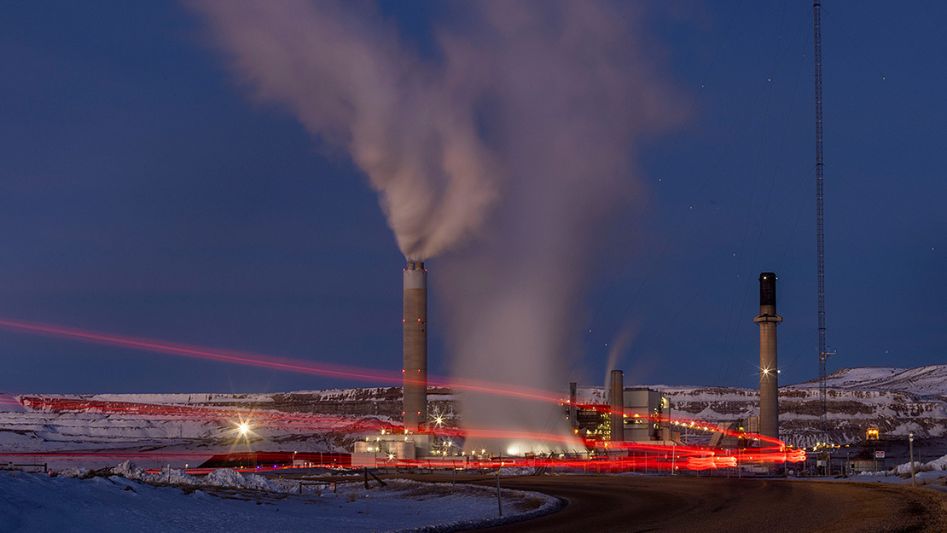
Nuclear engineer Jose Reyes, co-founder of NuScale Power in Portland, Oregon, claims that his company and its peers can bring the industry back from the brink by starting small. Small modular reactors (SMRs) were developed by NuScale and its 350 workers, led by Reyes, and would need just one percent of the area required by a conventional reactor. Each NuScale SMR would produce 60 megawatts of electricity, while a commercial reactor can produce 1 gigawatt. NuScale plans to build a power plant out of as many as twelve SMRs for roughly $3 billion.
However, bigger isn’t better in every case. Reyes, 63, a mild-mannered New York City native and the son of Honduran and Dominican immigrants, says, “If I merely scale down a massive reactor, I’ll lose, no doubt.” Engineers at NuScale have streamlined their reactors to make them safer, claiming that by doing so they have made them practically immune to meltdown by doing away with pumps, valves, and other moving components. To make their reactors competitive with other types of energy, the engineers aim to create them in their entirety in a factory rather than assembling them at a construction site.
Since its inception in 2007 at neighboring Oregon State University (OSU), NuScale has invested over $800 million in its design, with $288 million coming from the Department of Energy (DOE) and the remainder coming mostly from NuScale’s principal investor, the global engineering and construction giant Fluor. The design is now being licensed by the Nuclear Regulatory Commission (NRC), and the business has secured its first client, a utility association planning to begin building a plant in Idaho by 2023.
However, NuScale is not alone. The firm is benefiting from a worldwide upswing in interest in SMRs since comparable projects are being developed in China and Russia. According to Robert Rosner, a physicist at the University of Chicago in Illinois who co-wrote a paper on SMRs in 2011, “SMRs as a class have a potential to revolutionize the economy.” To our knowledge, NuScale is the only US-based firm actively pursuing SMR licensing and construction. Rosner thinks positively about the company’s future. Rosner believes that “NuScale has really made the case” that they will be successful.
The NuScale reactors are currently just virtual prototypes. The firm, however, has constructed a life-size replica of the reactor’s top in an industrial district to the north of the city. The gray cylinder, which is adorned with pipes and measures 8 meters in height, is not quite diminutive. It seems like the submarine’s control room has broken through the surface and is resting on the dusty land. Ben Heald, a reactor designer at NuScale, says they constructed it to test whether or not employees could fit inside for inspections. “It’s a fantastic promotional instrument.”
However, not everyone is certain that NuScale can become a functioning product. There have been dozens of different attempts at creating a more sophisticated nuclear reactor, and they have all failed. Allison Macfarlane, professor of public policy and geologist at George Washington University in Washington, D.C., and chair of the NRC from 2012 to 2014, says that even if NuScale and other startups are successful, the nuclear industry will not build enough plants quickly enough to matter in the fight against climate change. “Nuclear does not accomplish anything rapidly,” she explains.
Twelve packs of power
To create a NuScale facility, 12 compact modular reactors would be placed in a single pool of water. The basic design removes the pumps and pipelines that may fail and cause an accident, and each reactor contains passive safety systems that would help prevent a meltdown. The reactors would be assembled at a facility and sent to the site as a complete unit.
Nuclear power plants are just large, expensive boilers. Typically, pellets of uranium oxide are housed inside the fuel rods that hang horizontally within their central core. In a process known as fission, radioactive uranium atoms spontaneously break apart, producing energy and neutrons that go on to divide further uranium atoms. The heat from the reaction boils water, which turns steam turbines, which provide power.While there is a wide range of different designs (for more information, see the sidebar), the majority (around 85%) of the 452 power reactors in the world use water circulation through the core to cool it and transport heat to a steam generator that powers a turbine.
Water provides an additional layer of protection. The fissile uranium-235 isotope is normally present in only trace amounts in the fuel used in power reactors. Only by slowing the neutrons to improve the likelihood that they will split additional atoms can the diluted fuel keep up a chain reaction. The neutrons’ rate of decay is slowed by the cooling water itself. A runaway chain reaction like the one that blew up a graphite-moderated reactor at the Chernobyl Nuclear Power Plant in the Ukraine in 1986 may be prevented if that water is lost in an accident, rendering fission ineffective.
However, heat from the radioactive decay of nuclei released by fission may melt the core even after the chain reaction has died out. Unfortunately, this is exactly what occurred at Fukushima when the tsunami knocked out the backup power systems that were pumping water into the reactors.
The architecture of NuScale helps mitigate these dangers in a number of ways. To begin, far less decay heat would be produced by the tiny cores in the event of an accident. Engineers at NuScale have eliminated pumps that circulate cooling water through the core, instead relying on convection. An engineer with NuScale, Eric Young, explains that their design precludes the need for any moving components that may otherwise malfunction and cause an accident. If it isn’t there, he adds, it can’t be broken.
The redesigned reactor casings developed by NuScale provide an extra layer of security. A typical reactor is housed within a containment vessel made of reinforced concrete and measuring up to 40 meters in diameter. Each NuScale reactor, measuring 3 meters across, fits snugly within a steel containment vessel measuring 4.6 meters across, which can sustain pressures 15 times higher because of its reduced diameter. NuScale’s last line of defense, the sunken ships, form a large body of water.
In the event of an emergency, for instance, operators may use the pool’s heat exchangers to redirect steam from the turbines and chill the core. In normal operation, a vacuum is maintained between the reactor and the containment vessel to insulate the core and speed up the heating process. However, if the reactor were to overheat, relief valves would open, releasing steam and water into the vacuum space, where they would warm the pool. According to Reyes, such passive safety measures would keep the core intact in almost any kind of collision.
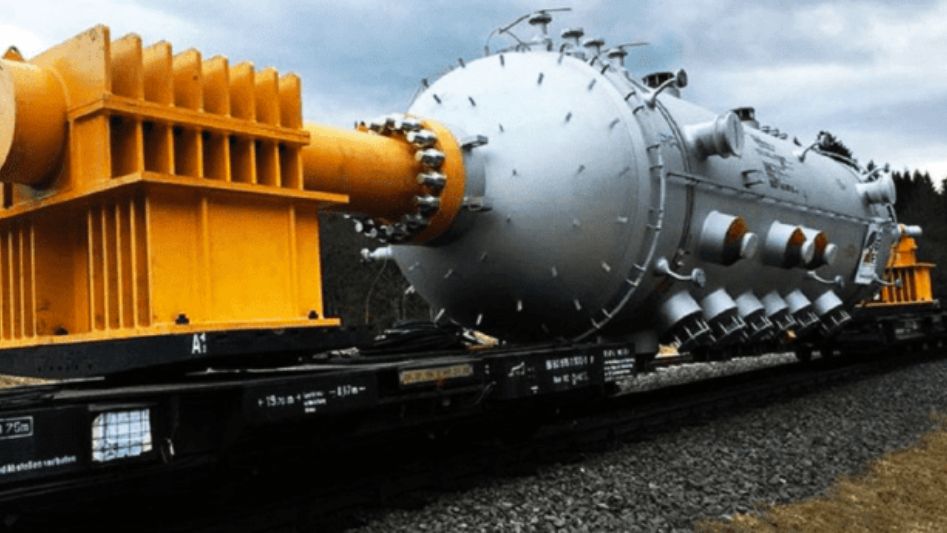
Engineers at NuScale have built a model at a third of the actual size to ensure the reactor would perform as expected. In the back of a nuclear engineering lab at Oregon State University is a 7-meter tower of pipes, valves, and cables. According to Young, the model’s primary purpose is not to replicate the performance of the actual reactor but rather to verify the accuracy of the computational models that the NRC would use to assess the design’s security. According to Young, the model’s core uses 56 electric heaters, similar to those used in curling irons, to warm water rather than nuclear fuel. “It’s like a giant percolator,” he explains. Over the course of three days, we put up a test to observe the coffee-making process.
According to M. V. Ramana, a physicist at the University of British Columbia in Vancouver, Canada, there is a drawback to shrinking a reactor. He claims that the higher running expenses will result from a smaller reactor’s inability to get as much power out of each tonne of fuel. The expansion of reactor sizes “has its justification,” Ramana explains. The benefits of scale are something that NuScale is inherently giving up.
Yet, Reyes argues that despite their small size, tiny vehicles are surprisingly flexible. Even just one small reactor might provide enough energy to run a desalination plant or heat an industrial operation. Adapting a NuScale plant to the needs of a developing country’s smaller electrical system is a real possibility. Even in the industrialized world, where the use of intermittent renewable sources like windmills and solar panels is on the rise, a complete pack of 12 reactors could offer reliable electricity. It is possible for a NuScale plant to “load follow” and fill up the gaps by adjusting the number of reactors providing electricity, as explained by Reyes.
Such ideas hint at another critical part of the NuScale plan: the designers’ desire to radically alter the structure and management of nuclear power facilities. The Nuclear Regulatory Commission mandates that every control room handling more than two reactors must employ at least six personnel. NuScale is requesting authorization to operate twelve of its simpler, safer reactors from a centralized control center. Ross Snuggerud, senior operations engineer at NuScale, says, “Employees have laughed at me when I stated I could manage this facility with six people.”
NuScale engineers proved the concept by creating a working control room for a digital power plant. NuScale’s control room is located on the second floor of its facility in an industrial park along the Willamette River, and it has a wall of massive, high-resolution monitors that show the status of the company’s 12 virtual reactors. Snuggerud used a touch screen on a recent day to simulate a catastrophe. One of the 12 simulated reactors experiences a surge in reactivity. The boron carbide control rods that are supposed to fall into the core to absorb neutrons and halt the reaction do not react as expected.
A buzzer sounds, indicating the presence of an imminent threat. There’s a brief flurry of illumination. The center heats up rapidly. However, the NuScale reactor appears unfazed by the situation. The reactor automatically diverts heat into the pool, causing temperatures to drop within minutes. So, can’t the core be melted if we try? Snuggerud asserts, “No responsible engineer would reply “never.” However, we have taken several precautions to safeguard the integrity of the core.
Engineers from NuScale need to persuade the Nuclear Regulatory Commission that a real plant will function as smoothly as their simulation. The application was filed two years ago by the corporation and has 12,000 pages; the evaluation process is expected to be completed by September 2020. In this regard, the NuScale staff is well-versed. Reyes assisted the NRC with the certification of two standard Westinghouse designs when he was a student at OSU. The NuScale design would be the first licensed by the NRC since 2014.
According to NuScale’s licensing expert, Carrie Fosaaen, the company has replied to more than 1,500 official requests for further information, which is roughly a third of the normal amount. “What we put together in the beginning really paid off,” she recalls. Fosaaen maintains, “Our design is so unusual that it’s a struggle even for individuals who have done a lot of licensing.”
Fosaaen argues that a literal reading of NRC rules would force NuScale engineers to construct a scaled-down version of a conventional reactor, which is the opposite of their goal. Her advice is to keep the NuScale design as simple as possible while convincing authorities of its safety.
It’s fair to say that NuScale is making some audacious demands. Given that the company’s reactors are designed to shut down in the absence of electricity, it has petitioned the NRC to waive the need for backup generators. Similarly, NuScale opposes having its reactors accompanied by a 32-kilometer-wide evacuation zone since, according to the company, there is little danger of radiation spreading beyond the plant’s boundaries. A utility might replace an outdated coal plant in a populous location with a NuScale facility if the rules were altered to allow for this. According to Reyes, “that’s something utilities actually desire.”
Such demands, according to one prominent critic, reek of arrogance. Edwin Lyman, a physicist with the Union of Concerned Scientists in Washington, D.C., claims that NuScale is removing layers of security from nuclear power plants in order to save money. It’s “simply unsafe and irresponsible” to claim “you know so well how a new reactor will function that you don’t need an emergency evacuation zone,” he warns. Nonetheless, a nuclear engineer from MIT in Cambridge named Jacopo Buongiorno has deemed NuScale’s proposals to be fair and plausible, suggesting that the company would likely be granted them. He argues, “I disagree that safety elements are being removed.” To the contrary,
The engineers at NuScale can’t wait to get started on a real plant. The business has a preliminary arrangement with Utah Associated Municipal Electricity Systems (UAMPS), a coalition of 46 municipal utilities in six western states, to develop a 12-pack plant at DOE’s Idaho National Laboratory near Idaho Falls as part of UAMPS’s carbon-free power initiative. One module would be used for research at Idaho National Laboratory, the Department of Energy’s (DOE) primary nuclear energy research facility, while the other would be used to provide electricity for the facility. If the remaining 10 modules were to be connected to the grid, then the grid would be fed. The UAMPS should make a decision on the plant this year, with construction beginning in 2027.
NuScale anticipates a domino effect from new clients. “There are many organizations that don’t want to be first but would definitely prefer to be second in line,” says Tom Mundy, chief commercial officer of NuScale. By 2035, small modular reactors (SMRs) might generate 65–85 gigawatts of electricity worldwide, a construction binge costing between $320 and 510 billion, according to a paper from the National Nuclear Laboratory in Sellafield, United Kingdom, published in 2014. Technical experts in Argentina, China, Russia, and South Korea have all created SMR models. Rosner believes that “internationally, NuScale is likely to be a competitive rival” due to the high quality of its design.
NuScale will have to contend with low prices for natural gas if it wants to be successful. to produce electricity at a total cost of $65 per megawatt-hour (including building and running the facility). In comparison to the present price of energy from a gas-powered plant, it is a 20% increase. However, according to Rosner, “the price of gasoline isn’t going to remain low forever.” The cost of fossil fuel electricity would increase if countries imposed a price on carbon emissions. In reality, a carbon price might make nuclear energy competitive with gas, according to a paper published by MIT in September 2018.
Ramana argues that if renewable energy sources like wind and solar power continue to decrease in price, nuclear power may face even more competition. If the Department of Energy (DOE) were to finance the transactions for NuScale in the same way that it has subsidized UAMPS, Lyman believes the company would still have trouble finding clients. He claims, “The economics are so awful, I simply don’t see this wave of little reactors across the globe.” Reyes, echoing the sentiments of many energy experts, believes that a renewables-based energy system would need a reliable source of “baseload” electricity; unlike natural gas, nuclear power can provide this without producing carbon emissions.
Despite NuScale’s best efforts, it may be necessary to go outside American shores to gauge the company’s long-term viability. The United Kingdom plans to shut down its seven remaining coal-fired power stations by 2025 in an effort to cut carbon emissions. Although the UK government has the option of switching to gas-fired plants in their place, NuScale is lobbying for the adoption of nuclear power. According to Mundy, “we are not a concept, and we are not a technology that is still in the planning stages.” It’s true; we’re here. We should know in a few years whether that is the case.
Conclusion
During normal operations, nuclear power plants don’t add to the amount of greenhouse gases that are released into the air.However, over the course of their entire lives, nuclear power plants emit about the same amount of carbon dioxide-equivalent emissions per unit of electricity as wind power and about one-third of the emissions that solar power contributes per unit of energy.
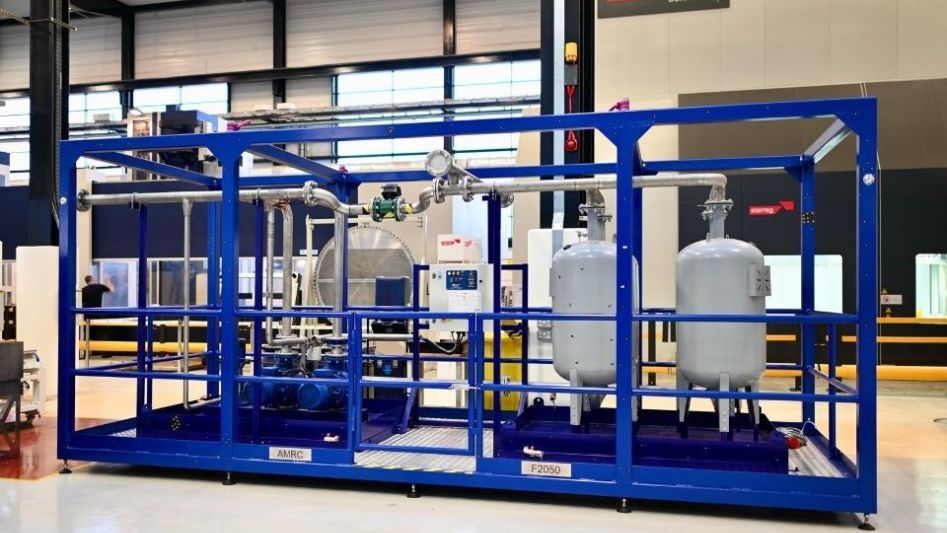
FAQ
To what extent may nuclear power mitigate global warming?
More than 470 million metric tons of carbon dioxide emissions are prevented annually thanks to nuclear-generated power.
When it comes to saving the globe, might nuclear power be the answer?
Because of its dependability and scalability, nuclear power can be used to directly replace fossil fuel plants, eliminating the need to burn fossil fuels to generate electricity. Nuclear power now in use prevents emissions about equal to taking 300 million automobiles off the road.
Why aren’t there any nuclear power plants in orbit?
Radiative thermal generators (RTGs) provide a unique challenge for nuclear power in space because of the necessity to dispose of waste heat. Unless you’re quite distant from the Sun, you’ll be stuck with heat-radiating panels instead of solar panels, which provide far less energy per kilogram.
If nuclear power were to be used, could it solve the energy crisis?
If you’re concerned about waste and security, you won’t find much solace in the numbers. Nuclear energy’s lengthy development timeline also fails to impress when compared to the time it takes to create renewable sources, which may be measured in months rather than years.
You May Also Like
- WIND ENERGY: PROS AND CONS
- HOW DOES A SMALL NUCLEAR REACTOR WORK?
- THE NEW HYDROGEN PRODUCTION METHOD NO-ONE IS TALKING ABOUT
- THE ‘SUPERHYBRID’ PROJECTS IN AUSTRALIA HELP WITH HYDROGEN GENERATION
- WHAT IS WIND ENERGY? DEFINITION, TYPES AND MORE