Generation IV reactors are intended to have better fuel efficiency, use recycled uranium, thorium or plutonium fuels, operate at very high burn up to reduce radioactive spent fuel waste.
Table Of Content
- The Cost of Nuclear Power
- Future reactor designs
- Small Modular Reactor (SMR)
- High-temperature gas-cooled reactor (HTGR)
- Gas-Cooled Fast Reactor (GFR)
- Sodium Fast Reactor (SFR)
- Lead-Cooled Fast Reactor (LFR)
- Fluoride-Cooled High Temperature Reactor (FHR)
- Molten Salt-Fueled Reactor (MSR)
- Beyond Gen IV
- Conclusion
- FAQ
Despite the ongoing debate, a surprising number of new nuclear reactors are being constructed, making nuclear power the second biggest source of carbon-free energy worldwide. Moreover, this is a sector that is always evolving as new technologies emerge. The future of nuclear power: what can we expect?
In the basement of the University of Chicago’s Stagg Field football stadium on December 2, 1942, the world’s first nuclear reactor, known as Chicago Pile 1 (CP-1), was turned on. Since then, a total of 440 reactors have been built, each producing enough power for over 10 percent of the global population.
Regardless, nuclear energy has a terrible reputation. This is the result of a confluence of forces, like so many other things in life. Many individuals continue to lack understanding about nuclear power. It’s stigmatized due to its association with nuclear weapons, the lingering effects of Cold War propaganda, and three high-profile reactor accidents in the United States, the Soviet Union, and Japan.
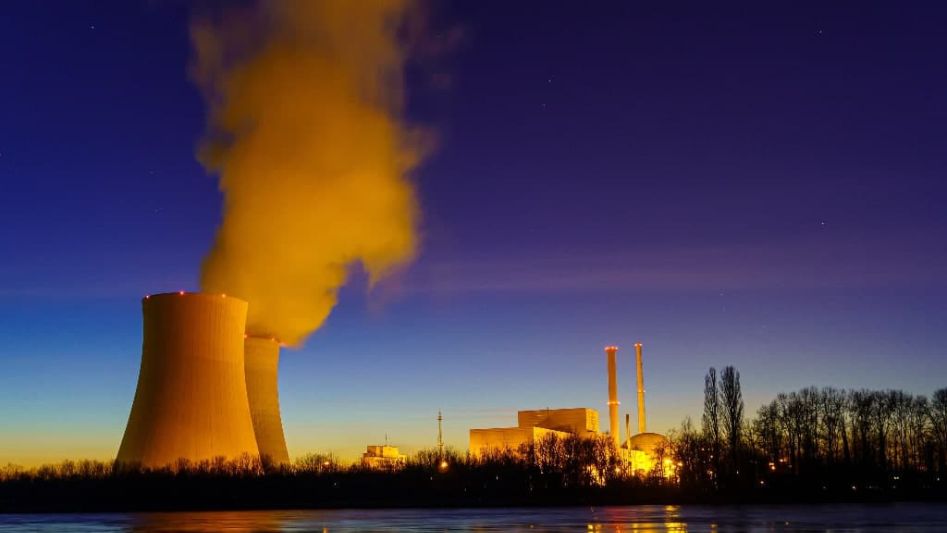
Western reactor research and building ground to a halt in the late 20th century, but the sector may be on the cusp of a resurgence. While nuclear energy has a bad connotation, it really offers certain positives. It produces no carbon dioxide or other greenhouse gases and has no other emissions. It generates enormous quantities of energy while taking up hardly any room at all. It is flexible in terms of location. In addition, it has the lowest fatality rate per kilowatt of any major energy source.
The Cost of Nuclear Power
Still, the expense of nuclear power is a major drawback. Considering the high cost of building a reactor (up to US$15 billion), the return on investment is usually not worth it. Instead, the majority of the contractor’s income comes from reactor maintenance and fueling.
Building a nuclear power station is expensive not because nuclear power is inherently expensive but because these civil engineering projects are rare, big, and may take up to 20 years to complete. Plants are assembled in the field rather than in a factory. The operator must shoulder all waste disposal expenses and go through a lengthy licensing procedure in which the plant’s design is tested, changed, and retested under a distinct set of quality, safety, and security regulations.
This not only causes budget overruns, but it also provides enough opportunity for the expertise of retiring engineers to be lost. Because of this, even countries like Britain, which was an early innovator in nuclear power, had to go elsewhere for assistance in constructing its newest reactors.
Costs may be reduced in a variety of ways, including the use of standardized designs, the construction of sufficient numbers of plants to provide continuity of expertise, the use of different management streamlining methods, and, most crucially, direct assault on the largest building cost. The most expensive parts of these cutting-edge systems are not the nuclear reactor and turbine islands themselves, but rather the surrounding infrastructure (such as roads, buildings, and the installation of electricity and other electrical services).
New reactor designs, some of which have been in the works for decades, are thus being looked to by the nuclear industry as a means to cut construction and operating costs, increase safety and efficiency, and lessen the danger of nuclear weapons proliferation.
Future reactor designs
The nuclear power sector is now operating in Generation III+. Prototype reactors from the 1940s through the early 1960s defined the first generation, while the first commercial light water reactors were built from the mid-1960s through the mid-1990s. Generation III followed, again consisting of light-water reactors but with upgraded features such as passive cooling systems and fail-safe reactor cores. By the 2030s, construction will have concluded on the newest Generation III+ reactors, which are essentially upgraded versions of Generation III designs.
Generation IV will be the next generation of nuclear power plants, and it will be a family of far more complex and diversified designs with the goal of making nuclear power plants cheaper and safer by combining new reactor technologies, new materials, and new manufacturing procedures.
Water, helium, liquid metal, or molten salt are all possible coolants for these Gen IV reactors. The region of the neutron spectrum at which they shine also serves to categorize them. meaning either the slow neutron spectrum or the rapid neutron spectrum. The former employs a moderator to delay the reaction, which takes place at lower neutron energies, whereas the latter works at extremely high neutron energies since the neutrons that produce fission are created by the nuclear reaction and are not slowed down.
Let’s have a look at some fourth-generation reactors. Not all possible candidates have been included, but those that have a good chance of becoming prominent by the middle of the 21st century are.
Small Modular Reactor (SMR)
Although the first nuclear submarines were powered by small reactors like those used in Small Modular Reactors (SMRs) in the 1950s, these reactors do not meet the World Nuclear Association’s definition of SMRs. However, the new SMRs being introduced today are so advanced that they sit in a gray area next to Gen IV reactors. Light water Small modular reactors (SMRs) are essentially improved versions of the reactors in use today; however, they are smaller and can be mass-produced like automobiles. These seek to use factory production practices in the nuclear energy sector in order to reduce associated costs. Small, standardized reactors with an output of less than 300 MWe are the core concept.
Small modular reactors (SMRs) are not massive civil engineering projects that may take twenty years to come online and another twenty to earn a profit. To the contrary, small modular reactors (SMRs) are built on a modular architecture that separates not only the reactor but also the majority of the auxiliary equipment.
This paves the way for power plants to be constructed as sturdy modules in factories or shipyards and then transported to the site for final assembly. One of the goals is to drastically reduce the time and money needed to get a plant up and running.
An additional perk of SMRs is their flexibility in plant layout to accommodate a wide range of client requirements. Facilities with numerous reactors may provide energy to millions, while smaller, more remote towns can purchase single-reactor plants to power, say, a few thousand homes and businesses. SMRs are useful for niche industries like oil exploration and military base support due to their compact size. Further, modules may be made to be transported by whichever methods are most practical, whether it is by barge, ship, truck, railway, or even airship.
Passive safety devices, such as those used to provide cooling in the event of an accident, are another distinguishing feature of SMRs. They can also be simply deployed underground or on ships or sea platforms where they lie below the water line, shielded in the same manner as the reactor on a submarine, eliminating the need for enormous concrete structures.
High-temperature gas-cooled reactor (HTGR)
A graphite-moderated helium-cooled reactor, a High Temperature Gas-Cooled Reactor (TGR), may reach temperatures twice or three times higher than those of a normal reactor while producing just a fraction of the power. Conceptually, this has been around since the 1940s, but only in the last few years has the technology caught up to the promise of the idea.
The HTGR is powered by tri-structural isotropic (TRISO) particles, which form its foundation. TSRIO fuel is composed of poppy-seed-sized particles of uranium, carbon, and oxygen enclosed in three layers of carbon or ceramic materials in order to confine nuclear waste products, as opposed to rods.
These fragments may take the shape of either spherical pellets or pebbles about the size of billiard balls. As a result, the gasoline is now extremely durable.It can withstand higher temperatures, oxidation, neutron irradiation, and corrosion than regular fuels. Since the pebbles will remain stable at greater temperatures, the reactor’s operating range is expanded. Additionally, the pebbles may be gently circulated throughout the reactor, with used stones being removed from the bottom and replaced by new pebbles inserted at the top.
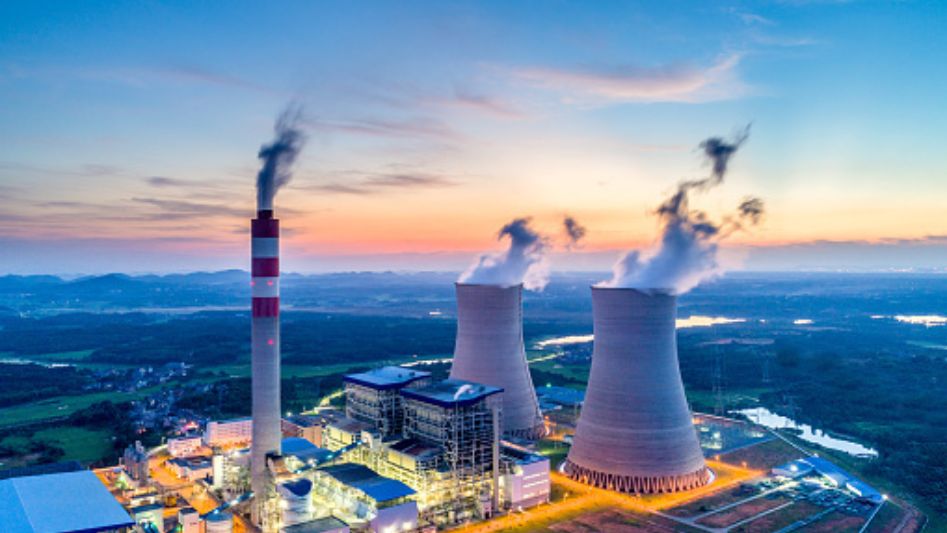
Gas-Cooled Fast Reactor (GFR)
Helium is used to cool the core of gas-cooled fast reactors (GFRs), which produce more power per unit volume than HTGRs. In contrast to conventional reactors, which create slow neutrons, fast neutron reactors transform thorium or non-fissile uranium isotopes into plutonium or fissile uranium isotopes, producing more fuel than they consume in the process.
A ceramic uranium mono-carbide fuel core is used in the GFR’s most sophisticated iterations, allowing them to function at very high temperatures. And the fuel is set up in such a way that there are many uranium atoms packed into a little space.
Sodium Fast Reactor (SFR)
The Sodium Fast Reactor (SFR) is another kind of fast reactor; it is cooled by liquid sodium, which is excellent at dissipating heat. Because bigger sodium reactors are inherently unsafe, these smaller reactors may use passive and inherent safety measures. While uranium oxide fuels are preferred in Russia, France, and Japan, uranium-zirconium alloys encased in steel are employed in the United States. Due to the low thermal density of these fuels, the nuclear reaction will automatically shut down if the reactor core becomes too heated.
Because the SFR has a closed fuel cycle, the core is likewise relatively small. Therefore, the reactor may operate for decades without needing to be refueled, since the uranium and plutonium are recycled inside the core as part of the nuclear reaction.
Lead-Cooled Fast Reactor (LFR)
Based on a reactor design created for Russian nuclear submarines, the lead-cooled fast reactor (LFR) employs lead as its cooling element. More recent models use uranium nitride as fuel rather than uranium dioxide. Lead, like sodium, may operate as a passive safety device to automatically rein in an uncontrolled nuclear event.
Fluoride-Cooled High Temperature Reactor (FHR)
Reactors that operate at high temperatures but are cooled by a molten salt combination of lithium fluoride and beryllium fluoride are known as fluoride-cooled high-temperature reactors (FHR). Utilizing TRISO-particle fuel technology, these reactors may produce up to ten times the power density of a conventional HTGR. For future designs, pebble fuels will be used, and the use of fluoride salts will enable the reactor to operate at lower temperatures than helium-cooled reactors.
Molten Salt-Fueled Reactor (MSR)
The molten salt in the MSR serves as both a coolant and a fuel source. The fuel is not shaped into rods, pellets, or pebbles but rather mixed with the fluoride salt, which then runs through graphite or a similar moderator that produces slow neutrons and regulates the process.
Although MSRs may function at higher temperatures, doing so can cause corrosion; hence, cooler versions are often favored in design. The combination of the coolant and the fuel, however, makes waste removal and fuel replacement simpler than in traditional reactors.
Beyond Gen IV
These Generation IV reactors will start operating as the need for carbon-free electricity spurs the construction of additional nuclear power stations throughout the globe. They are expected to gain popularity quickly due to their low production costs and quick assembly time.However, what comes next is a mystery. I’m curious about the characteristics of the next generation, Generation V.
We should expect to see new nuclear facilities for new niche uses, but in many respects they will be upgraded versions of the Generation IV reactors, building on the lessons learned from the preceding generation. Small reactors for use on the moon are already in the works, as are related technologies like nuclear fuel that burns like a candle, with the reaction beginning at one end and progressing to the other as the fuel is consumed.
Other methods of nuclear reactor design that were based on trials performed decades ago but were discarded in favor of more promising alternatives may potentially be revived. Experts in the area may only have a vague knowledge of some of them since they were abandoned for so long. They’re being reviewed again right now. Maybe one day thorium will be as commonly understood as uranium and plutonium, and the word “nuclear fuel” will include all of these elements.
No one can say for sure that nuclear fission won’t go the way of the coal-fired locomotive if nuclear fusion is ever proven viable.
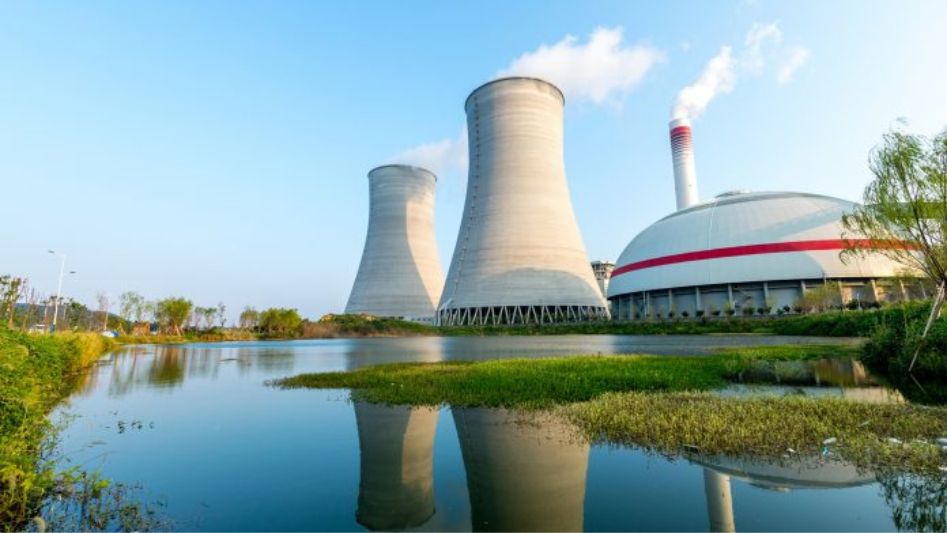
Conclusion
In comparison to GEN III facilities, the potential uses of GEN IV technology (middle- and high-temperature co-generation, electricity production, actinide management, energy supply for isolated grids, and others) are extensive. However, the “carbon cost” for fossil-fired power plants would raise their relative worth, making GEN IV NPPs more expensive than present LWRs. To be commercially viable, GEN IV NPPs will need much more research and development time.
FAQs
Why is the future of nuclear power generation considered bright?
In reality, nuclear power accounts for more than half of America’s zero-carbon electricity. Carbon emissions from nuclear power plants are reduced by more than 100 million cars per year. When it comes to energy generation, nuclear power plants are by far the most dependable option.
What is 4th generation nuclear power?
Generation IV nuclear power refers to a potential solution to the issues that have plagued the nuclear power industry up to this point: a network of reactors and nuclear fuel cycle facilities, including fuel fabrication plants and reprocessing facilities.
What are the main characteristics of Generation IV nuclear?
Nuclear waste that stays radioactive for a few decades as opposed to millennia is produced by Gen IV reactors. With the same quantity of nuclear fuel, we can produce 100–300 times as much energy. increased variety of fuels, including those that haven’t been refined or refined to a refined state (non-pebble MSR, LFTR).
You May Also Like
- CAN SMALL NUCLEAR REACTORS SAVE US FROM A WARMING PLANET?
- HOW DOES A SMALL NUCLEAR REACTOR WORK?
- TOP COUNTRIES WITH HIGHEST PROPORTION OF RENEWABLE ENERGY
- HERE ARE THE BATTERIES THAT COULD POWER THE FUTURE
- ENERGY: WHAT DOES THE FUTURE LOOKS LIKE?